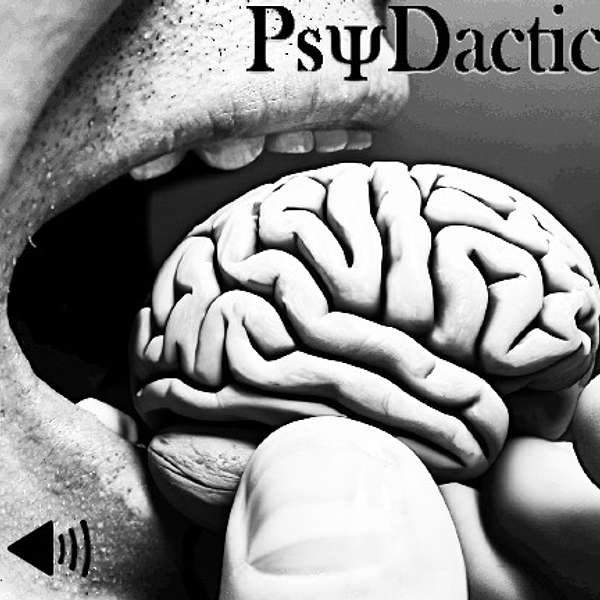
PsyDactic
A resource for psychiatrists and other medical or behavioral health professionals interested in exploring the neuroscientific basis of psychiatric disorders, psychopharmacology, neuromodulation, and other psychiatric interventions, as well as discussions of pseudoscience, Bayesian reasoning, ethics, the history of psychiatry, and human psychology in general.
This podcast is not medical advice. It strives to be science communication. Dr. O'Leary is a skeptical thinker who often questions what we think we know. He hopes to open more conversations about what we don't know we don't know.
Find transcripts with show-notes and references on each episodes dedicated page at psydactic.buzzsprout.com.
You can leave feedback at https://www.psydactic.com.
The visual companions, when available, can be found at https://youtube.com/@PsyDactic.
PsyDactic
Extrapyramidal Side Effects and Tardive Dyskinesia for Super Nerds
This episode explores side effects of antipsychotics at the molecular level. It starts by exploring receptors and their ligands and takes a turn into the dorsal striatum where dopamine, acetylcholine, serotonin, and glutamate work together to help us dance the mamba. Dr. O'Leary explores what happens when the complex pathways of the cortico-striatal-thalamo-cortical tract are disrupted by antipsychotics both in the short term and after many months or years of use. As the title suggests, there is a ridiculous amount of detail, so let's get our nerd on!
Please leave feedback at https://www.psydactic.com.
References and readings (when available) are posted at the end of each episode transcript, located at psydactic.buzzsprout.com. All opinions expressed in this podcast are exclusively those of the person speaking and should not be confused with the opinions of anyone else. We reserve the right to be wrong. Nothing in this podcast should be treated as individual medical advice.
Welcome to PsyDactic Residency Edition. I am Dr. O’Leary and today is Wednesday, December 13, 2023. This is a podcast primarily about psychiatry and neuroscience. I am currently a 4th-year psychiatry resident and I make this podcast as a way to structure my own learning. Having a task to accomplish focusses me and makes me question and double check myself. I don’t have an editorial staff to help me and this podcast is no one else’s opinion. That includes the federal government, department of defense, defense health agency, or the Dill Pickle Club.
A couple of episodes ago, I promised a more in-depth look at antipsychotic medications, also known as neuroleptics, and originally called “major tranquilisers.” However, I got distracted by a new study claiming to have reanalyzed the STAR*D trial with very different results. Check out my last episode for this. Today, I am back on track exploring antipsychotics. I thought it would be an easy topic, but the more I read, the more rabbit-holes I discovered. Molecular biology and pharmacology can seem like impossibly dense collections of facts, and if approached this way, they certainly are. One thing that helps me is to approach it in an inside-out kind of way. If I can learn why all these disparate appearing facts matter, then I am much more likely to be able to remember each of them individually. The relationships between them improve my recall of them.
To understand antipsychotic and especially their side effects, I needed to review some pharmacodynamics. Pharmacodynamics is a word I use when I want listeners to get bored and skip the rest of the podcast, so if you plan to remain with me, welcome to the land of super-nerds.
Most of the doctors or student doctors listening will be familiar with the terms agonist and antagonist with respect to pharmacologic agents. An agonist is something that binds to a receptor on a cell and makes that receptor do more of something, like allow ions to flow, or start sending a signal, or send more signals than it does when it is just hanging out. An antagonist binds to a receptor and prevents an agonist from binding, effectively keeping the receptor from being stimulated. Importantly, an antagonist doesn’t change the basal firing rate or level of ion flow regulated by the receptor. It just prevents that rate from changing. An antagonist not only blocks an agonist but also blocks inverse agonists. What are inverse agonists, Dr. O’Leary? Well…
Let's back up and talk first about receptors themselves. There are many kinds of cell receptors, but they broadly do three different things. They either send a signal, open an ion channel, or become enzymatically active (which means their catalytic site is exposed and whatever reaction they facilitate and starts to function). The dopamine receptors fall roughly into two of these classes. They either affect ion channels or send signals.
For example, there are 5 basic types of dopamine receptors, called D1, D2, D3, D4, and D5. To help me remember what type of receptor each of these are, I think of a dopamine receptor sandwich. D1 and D5 are like the bread on the sandwich. They are very similar and are referred to as the D1-like receptors. D2, 3, and 4 are like the meats of the sandwich. They are often referred to as D2-like receptors. The bread, D1 and D5, when agonized increase the production of cyclic AMP which stimulates a pathway that ultimately leads to increase in transcription in the nucleus. These D1-like receptors ultimately result in signals to the nucleus to make mRNA that is translated into proteins.
The second group of receptors, or D2-like receptors, when agonized, reduce the production of cAMP. Instead of increasing transcription, these receptors modulate ion concentrations by (for example) activating potassium channels, which changes the excitability of the neuron. Often D2-like receptors, when stimulated, will reduce the firing rate of its neuron. This means that one of the functions of dopamine is to reduce the firing rate of target neurons. If a neuron with a lot of D2 receptors is being hit by dopamine, it will do less of what it normally does. Often these D2 neurons are gabaergic, and since gaba sends an inhibitory signal, that means less of the inhibitory signal is being sent. The overall result is that the target of the gabaergic neuron will fire more. Basically, by inhibiting an inhibitor, dopamine hitting D2-like receptors results in downstream disinhibition and greater activity of a neuron that it doesn’t directly modulate. Relationships like this are what make our brain capable of complex calculations.
In general, the D2 thru 4 receptors have a much higher affinity for dopamine than the D1 and D5 receptors. What this means is that the D2,3,4 receptors are more sensitive to small changes in tonic levels of dopamine while the D1 and D5 receptors will respond more during phasic dumps of dopamine, which happens when something is particularly salient or rewarding. On a molecular level, small changes in the level of dopamine will ultimately affect potassium channels through D2,3,4 stimulation, and fast, large changes in synaptic dopamine concentration can result also in transcription in the nucleus through stimulation of D1 and D5 receptors. Also, the relative abundance of D1-like and D2-like receptors are different on different neurons. For example, in the dorsal striatum there are separate D2 and D1 medium spiny neurons that respond very differently to dopamine. I’ll get back to that later.
For now, let me get back to the difference between an agonist and an inverse agonist. Many receptors have a basal rate of firing, which means that they are spontaneously active at some programmed rate. An agonist increases the rate or activity of the receptor. An inverse agonist, when it binds to a receptor, reduces the rate of firing or rate of activity and can shut it off entirely. It does the opposite of an agonist. An antagonist merely blocks the effects of agonists and antagonists, so when an antagonist binds, the basal rate of firing of a receptor is not changed. It will not increase or decrease. An antagonist prevents change. Agonists and inverse agonists result in change, but the change is in opposite directions.
Finally, there are partial agonists. A partial agonist is special because it competes with full agonists for the same receptors. It is called a partial agonist because, relative to the neurotransmitter it is imitating, when it agonizes a receptor, the increase in activity is less than what it would have been for the full agonist. If there is a low concentration of full agonists, then a partial agonist will have the overall effect of increasing the total activity of the receptors on the cell, but if the concentration of the full agonist is high, then a partial agonist will compete with it and reduce the sum total activity of the receptors on the cell. Partial agonists basically raise the floor and lower the ceiling of possible receptor activity.
I am going to go uber-super-nerd here, so if you weren’t sure you wanted to keep listening to this podcast, this is a good place to get off.
Some neurons, like those medium spiny neurons in the dorsal striatum, express different concentrations of dopamine receptors. There are D1-dense neurons and D2-dense neurons. Both can respond to bursts of dopamine, but the D2 will respond more to alterations in the tonic levels of dopamine. These neurons are part of a system that regulates the flow of glutamatergic inputs from the cortex, through a switch board consisting of neurons in the putamen, globus pallidus, and subthalamic nucleus that ultimately send signals to the thalamus, which then sends glutamatergic signals back to the cortex. In the middle there is a lot of gaba and dopamine, and these inputs are also regulated by acetylcholine and serotonin.
I did a show on this cortico-striatal-thalamo-cortical tract (I will call CSTC from here on out) way back in episode 8 on the 29JAN2022, and the episode before last I talked about the major dopamine pathways in the brain. If you want to review these first, I think it will help you going forward. I am going to get even more into the weeds now, in part because I can’t help myself, and also because I am taking an advanced psychopharmacology course. This CSTC is not just one circuit. There are multiple pathways including the motor circuit (which includes projections from the sensory motor cortex, the associative circuit (which includes projections from the DLPF and OFC), and the limbic circuit, which includes projections from the limbic regions. I am going to focus on the motor circuit because it is the easiest to see the effects of.
The motor side effects of antipsychotics (things like rigidity, parkinsonism, tremors, oculogyric crisis, akathisia and tardive dyskinesia) are a result of drug induced dysfunction primarily in the dorsal striatum. For me, it makes more sense to work backward through the CSTC loop. The last part of this loop consists of the thalamus sending glutamatergic (which are excitatory) signals back to the cortex. The whole point of this loop is to either shut off or allow these signals to get through. Depending on what you want to do, you may need to inhibit part of your motor cortex. For example, if you want to flex your arm, you can’t extend it at the same time. This loop makes this possible. One of the reasons our babies take so long to learn their motor skills is because this loop is constantly reprogramming itself during development.
The Globus Pallidus Interna acts as a gateway to the Thalamus, and the cells from the GPi are able to inhibit the thalamic projections or not. There are two main ways to get to the GPi. One is through the direct pathway. The other is through the indirect pathway.
The direct pathway goes… well… directly from the putamen to the GPi. Neurons of the direct pathway dump gaba onto GPi neurons which prevents them from dumping their gaba onto the Thalamus. Here is where we start getting deep into the double negatives. By inhibiting the the GPi which inhibits the thalamus, the thalamus is now free to send its load of glutamate back to the cortex. The direct pathway then is disinhibitory. In math, we would just reduce a double negative to a positive, but in the brain, having these double negative pathways is important to be able to fine-tune outputs. The direct pathway disinhibits the thalamus.
The indirect pathway is more complex. Before getting to the GPi, the indirect pathway goes through the subthalamic nucleus. The subthalamic nucleus sends out glutamatergic neurons to the GPi, which means that when these neurons fire the net effect is to reinforce inhibition of the thalamus. To do this, projections from the putamen first dump gaba onto neurons in the globus pallidus external (which is next to the interna). The neurons in the GPe are now inhibited and cannot deliver their gaba to the STn. The STn then is disinhibited allowing the subthalamic nucleus to send its glutamate to the GPi which then sends gaba to the Thalamus and inhibits output from the Thalamus reducing the excitatory signals to the cortex. The indirect pathway, then, can be described as dis-dis-inhibitory.
You could summarize everything by saying the direct pathway does things and the indirect pathway inhibits things, but simply understanding this is inadequate to understand the motor side effects of antipsychotics.
The neurons that carry gaba from the putamen along the direct or indirect pathway are bathed in dopamine by the substantia nigra pars compacta. The direct pathway is dense with D1 receptors and the indirect pathway is dense with D2 receptors. Antipsychotics tend to hit D2 receptors with a high affinity, especially the first generation antipsychotics. Dopamine has an overall dampening effect on target neurons, so blocking dopamine receptors will result in an overall derangement of the balance between the direct and indirect pathways. The result of this is aberrant signals being sent from the motor cortex, rigidity, torticollis, tremors, etc.
There are both pre-and postsynaptic D2 receptors that are blocked by antipsychotics. The presynaptic receptors are supposed to tell the neuron that there is enough dopamine so chill out and start cleaning it up, but if these receptors are blocked, excess dopamine will remain in the synapse. This dopamine is blocked from hitting D2 receptors so where is it going to go? More signals will then start to be sent through lower affinity or lower density dopamine receptor subtypes like D1 or D3.
But it gets much more complicated than that. Dopamine and glutamate are not the only performers in this delicate dance. Serotonin and acetylcholine both have roles to play. The same medium spiny neurons in the direct and indirect pathways that have dopamine receptors also have muscarinic acetylcholine receptors and are regulated by acetylcholinergic interneurons that themselves have D2 receptors. The acetylcholinergic neurons stimulate MSN to release gaba, and just like the MSN, their activity is downregulated by dopamine. This means that while dopamine directly reduces the activity of gabaergic MSN it also indirectly reduces their activity by reducing the activity of acetylcholinergic neurons. D2 blockers, like antipsychotics then would result in an excess of acetylcholine release.
The medical students and physicians in the audience will remember that we often give hefty doses of anticholinergic agents like diphenhydramine along with haloperidol when we need to emergently calm violent patients. The idea here is that by giving the D2 blocker, there will then be an excess of acetylcholine, and by blocking the action of acetylcholine, we can reduce the incidence of extrapyramidal symptoms. It used to be common practice to prescribe something like benztropine along with antipsychotics for chronic use in order to reduce the incidence of EPS, but this strategy was not very effective and also likely contributed to the kinds of cognitive impairment that anticholinergics can result in.
Young, healthy folks can withstand a lot of chemical insults to the brain, but people with dementia, like Alzheimers or Lewy Body disease or Parkinson’s disease are much more sensitive to antipsychotics. For Alzheimers in particular, patients are often given drugs like donepezil or galantamine and these medications inhibit acetylcholinesterase, the enzyme that breaks down acetylcholine in the synapse. As a result, when given with a D2 blocker that disinhibits acetylcholine release, it results in even more acetylcholine in the synapses. In the CSTC, this results in EPS, especially bradykinesia.
Remember that the MSNs are initially getting glutamatergic inputs from the cortex. Glutamate agonizes NMDA receptors and ultimately results in greater release of gaba by the MSNs. Dopamine shipped from the substantia nigra should tone this down, but if D2 receptors are blocked, it cannot. Another way to tone down the MSNs, since they are getting excited by glutamate without any way to counter this, is to give a NMDA receptor antagonist like memantine. Studies have shown that patients who have dementia and are on memantine are less sensitive to antipsychotics.
So blocking D2 receptors results in excess acetylcholine release onto MSN and unopposed glutamatergic input, both of which aberrantly increase gaba signally downrange and likely contribute to development of EPS. The strategy of adding on an anticholinergic was not very successful, and merely tacking on memantine can have other effects that we are not anticipating. For Alzheimer patients already on memantine, it is likely helping reduce EPS, but memantine does not have a clear role in treatment of psychosis. There is one more player that I haven’t gotten to yet. That player is serotonin.
The dorsal raphe nucleus is one of the places where serotonin is produced. Part of that serotonin is shipped to the striatum and the substantia nigra and helps to regulate the activity of the neurons there and even the release of dopamine. This means that there are serotonin receptors on all the neurons that I have mentioned above. In particular, the 5HT2a receptor is abundant on the dopaminergic neurons from the SN. If agonized, it reduced dopamine release.
Here is where the majority of the second generation or atypical antipsychotics come in. Not only are these neuroleptics D2 blockers, but they also block 5HT2a and often 5HT6 and 5HT3. They may also agonize 5HT1A. Here is how all of this is important. Antagonizing 5HT2a increases the release of dopamine from the substantia nigra to the striatum, and this will tone down the MSNs. Antagonizing 5HT2a along with 5HT6 on the acetylcholinergic neurons inhibits release of acetylcholine. Agonizing 5HT1a will reduce both glutamate and acetylcholine release into the striatum and also directly reduce GABA release by the MSN. Overall, their effects seem to counteract the effects of the D2 blockade and reduce the incidence of EPS and reduce the development of tardive dyskinesia.
I should reinforce that a reduced incidence is not no risk and long term use of any antipsychotic other than clozapine carries a risk of TD, and TD is what I am going to talk about next, but before I do I want to give oculogyric crisis a quick shout out. An oculogyric crisis results from abnormal contraction of the extraocular muscles. Most often I have heard it described as deviation of the cornea upward, like you are trying to stare at your own brain. This involves a distinct pathway in the striatum that bypasses both the direct and indirect pathway. Instead of stopping in the GPe, GPi, or STn, the gabaergic projections from the putamen reach all the way to the substantia nigra pars reticulata.
The pars reticulata is not like the pars compacta. The reticulata is gabaergic and it tonically inhibits regions in the thalamus and superior colliculus that themselves inhibit unnecessary movements, especially of the eyes. For example, when eyes need to perform seccades, projections from the direct pathway medium spiny neurons inhibit the gabaergic projections from the SNr to the superior colliculus which in turn allows the eyes to move together. Disruption of the function of this pathway is thought to be one of the causes of oculogyric crisis.
Now I can move on to Tardive Dyskinesia. TD is a drug induced movement disorder characterized by involuntary repetitive movements. It is most obvious in the face and tongue but not isolated there. Someone with tardive dyskinesia may excessively blink, purse their lips, make chewing like motions, move their jaw sideways, protrude their tongue, rock back and forth, extend their toes, etc. Obviously this can result in a lot of social stigma. Unlike most of the symptoms of EPS that can be present in other disease states or the result of brain injuries, tardive dyskinesia is exclusively a result of long term use of antipsychotics. Nothing else causes it. All antipsychotics can cause it except for clozapine and that is because clozapine barely touches the D2 receptor.
Tardive is called tardive because it is late onset. It is tardy, late to the game. The reason it is late is because it requires adaptations of neurons, especially the dopaminergic and medium spiny neurons. The first adaptation thought to play a role has to do with the number and responsiveness of D2 receptors. Because D2 is being consistently blocked, one of the things a cell can do to get more input from dopamine is to make more receptors. Another thing it could do is make the receptors more sensitive. Finally it could amplify whatever signal that receptor is sending. While there IS evidence that D2 receptor numbers are upregulated, it does not appear that the receptors themselves are hyperactive or their signals are amplified.
A few other things could happen. The presynaptic neurons can adapt as well. They could for example make and release more dopamine to counter the dopamine blockade. One way to do that is to upregulate the synthesis of dopamine and the vesicles that ship dopamine to the synapses. Another way to arrive at more dopamine in the synapse wouldn’t involve making more dopamine but simply to reuptake the excess of dopamine that is already in the synapse and repack that excessive dopamine into vesicles for release later. These vesicles are called vascular monoamine transporters type 2 or VMAT2s for short. There is evidence that this increase in packaged dopamine may contribute to TD, and the FDA has approved two VMAT inhibitors deutetrabenazine and valbenazine. VMAT inhibitors may also be prescribed for choreiform movements in Huntington's Disease. In the end, what VMAT inhibitors do is decrease the delivery not only of dopamine, but also of serotonin and norepinephrine to the synapse.
Another way to get a signal through when the signal receptors are blocked is to expand the surface area of a synapse. This appears to happen through adaptations of the presynaptic neurons. The contact area changes shape. It can have a kind of donut or horseshoe shape and possibly even split into what appears to be two separate synapses, with discontinuous areas between them. When observed in animal models these were termed perforated synapses because of their appearance. The process results in these adaptations has been called “maladaptive synaptic plasticity” because, in the case of TD, the end result is dysregulated signaling, both in the presents of and during withdrawal from D2 blocking agents.
So here is a summary of what giving D2 blockers chronically may do to result in TD. First, the number of postsynaptic D2 receptors will increase in number. The primary way to treat this is to keep someone on their antipsychotic because withdrawing them from it will result in severe worsening of their TD symptoms because there are so many more D2 receptors now. Second, more dopamine is made available, likely because it is not being used up, and so can be repackaged into VMAT2s and shipped back to the synapse. This can be treated by giving VMAT2 inhibitors, which will slow the release of not only dopamine but of serotonin and norepinephrine as well. Finally, the synapses have adapted, changed shaped, and increased their surface area. There is not much that can be done about that.
Because many of these changes are permanent, about a third of TD cases are irreversible. I encourage the listener to go onto YouTube and type in “living with tardive dyskinesia” and see what you find.
This episode is as long as it needs to be for now. In the future, I want to finally talk more about specific antipsychotics and which ones have higher rates of various kinds of side effects, for example how the affinity for the dopamine versus the serotonin receptors matter, or why partial agonists can appear to act like antagonists. Until next time, I am Dr. O and this has been an episode of PsyDactic Residency Edition.
Jones DG. Synaptic plasticity and perforated synapses: their relevance for an understanding of abnormal synaptic organization. APMIS Suppl. 1993;40:25-34. PMID: 8311991.
Ali Z, Roque A, El-Mallakh RS. A unifying theory for the pathoetiologic mechanism of tardive dyskinesia. Med Hypotheses. 2020 Mar 16;140:109682. doi: 10.1016/j.mehy.2020.109682. Epub ahead of print. PMID: 32200182.
Martel JC, Gatti McArthur S. Dopamine Receptor Subtypes, Physiology and Pharmacology: New Ligands and Concepts in Schizophrenia. Front Pharmacol. 2020 Jul 14;11:1003. doi: 10.3389/fphar.2020.01003. PMID: 32765257; PMCID: PMC7379027.
Ohno Y, Kunisawa N, Shimizu S. Antipsychotic Treatment of Behavioral and Psychological Symptoms of Dementia (BPSD): Management of Extrapyramidal Side Effects. Front Pharmacol. 2019 Sep 17;10:1045. doi: 10.3389/fphar.2019.01045. PMID: 31607910; PMCID: PMC6758594.
Reed MC, Nijhout HF, Best J. Computational studies of the role of serotonin in the basal ganglia. Front Integr Neurosci. 2013 May 24;7:41. doi: 10.3389/fnint.2013.00041. PMID: 23745108; PMCID: PMC3663133.
Solmi M, Pigato G, Kane JM, Correll CU. Treatment of tardive dyskinesia with VMAT-2 inhibitors: a systematic review and meta-analysis of randomized controlled trials. Drug Des Devel Ther. 2018 May 14;12:1215-1238. doi: 10.2147/DDDT.S133205. PMID: 29795977; PMCID: PMC5958944.